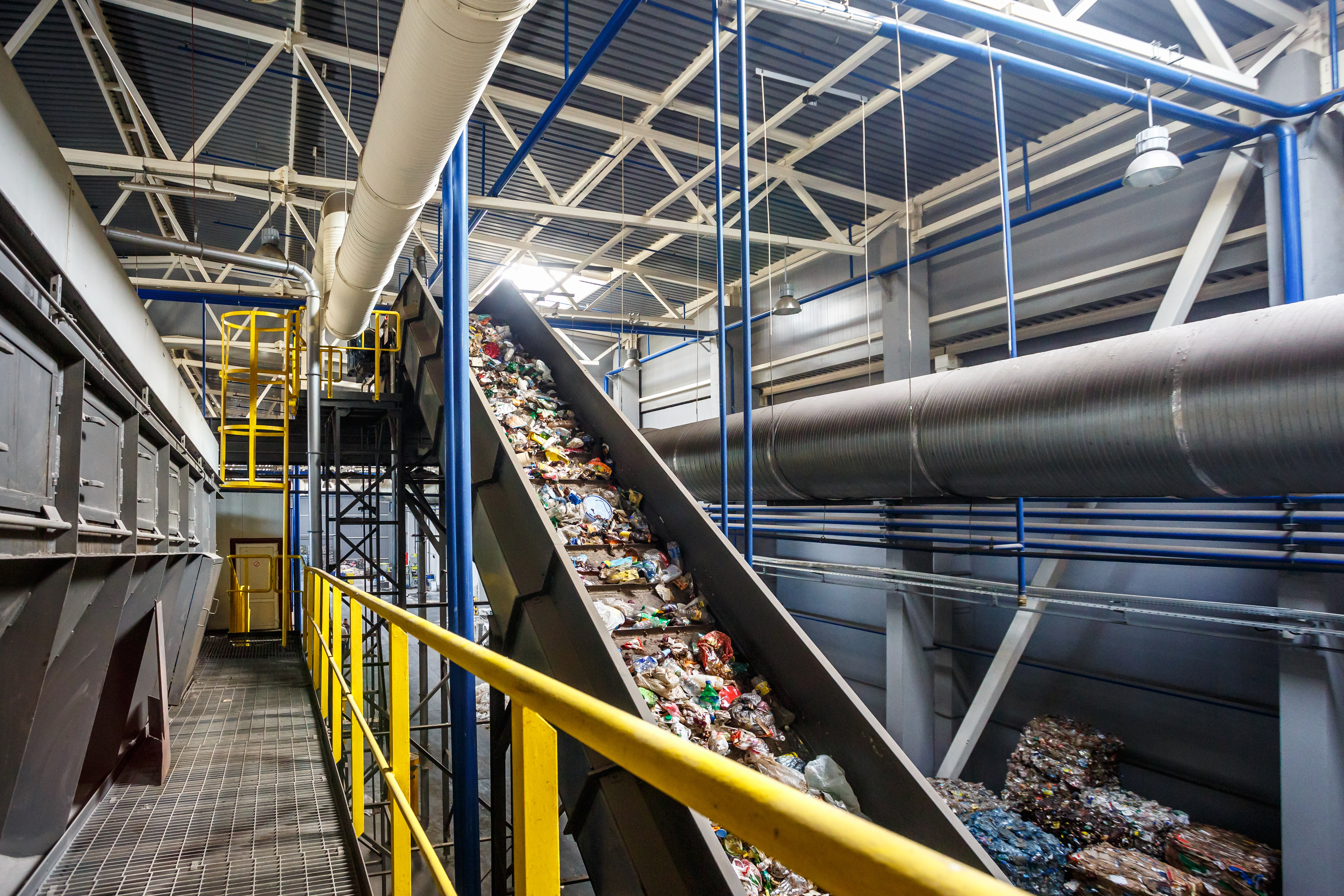
Back in 1979 The Buggles sang “video killed the radio star”. However, the radio(pharmaceutical) star is alive and burning hot. We provide an introduction to the area and touch upon some exciting upcoming developments in this post.
Radiopharmaceuticals, are medicinal compounds containing radioactive isotopes of atoms, known as radionuclides. They are used in the field of nuclear medicine for the research, treatment and diagnosis of diseases. In particular, radiopharmaceuticals are essential to the field of nuclear imaging, which includes techniques such as PET and SPECT (positron emission tomography and single photon emission computed tomography). Both of these techniques rely on the injection of a radiopharmaceutical, or tracer, in order to create image contrast.
Well-designed tracers accumulate preferentially at a target area. Any tracer which does not make it to the target is cleared from the body. The radiation released by the accumulated tracer passes through the body and can then be detected outside the body to create detailed 3D images of the target area. The specific targeting of the tracers to areas of disease makes these techniques incredibly powerful, revealing detailed and functional information about the specific organ or tissue without the need for invasive procedures.
One excellent example of a PET tracer is [18F]FDG. This is an analogue of glucose where one of the –OH groups is replaced with a radioactive isotope, fluorine-18. Metabolically hungry cancer cells rapidly take up this compound and phosphorylate it. The phosphorylation then prevents the molecule from leaving the cell and going back into circulation. At the same time, the placement of the fluorine-18 means that the [18F]FDG cannot be further metabolized by the cell, it thus becomes trapped. As a small and soluble molecule any [18F]FDG which remains in circulation is rapidly cleared through the kidneys meaning that after a short while, the majority of [18F]FDG in the body is trapped in cancerous cells allowing them to be highlighted with a PET scan.
Targeting is not restricted to metabolic differences like that exploited by [18F]FDG. The genome is the limit for the design of radiotracers. Useful biological targets which can produce an informative image of disease state are often referred to as biomarkers. These can include a whole range of proteins, many tracers aim to bind cell surface receptors, such as ion channels, inflammatory receptors and transporters. Alternatively, processes ongoing within the cell can be targeted, such as enzymatic processes or the expression of certain proteins.
Nuclear imaging is extremely useful in pathologies other than cancer too. Cardiovascular disease is commonly followed using radiotracers which image how blood flows through the heart, detect dying heart cells, or image atherosclerotic plaques. Neurological conditions are also an important area for nuclear imaging. In Parkinson’s disease for example, SPECT imaging with tracers that target dopamine transporters is used in diagnosis and disease progression monitoring.
While the radionuclide provides the signal required for imaging with tracers, it is usually the scaffold that dictates where in the body a tracer will accumulate. The scaffold could be anything from a small molecule (like [18F]FDG) to a radiolabeled peptide, protein or polynucleotide.
It is important that the properties of the radionuclide are matched appropriately with the tracer scaffold. For example, a radionuclide with a short half-life such as 18F (t1/2 ~2 hours) is most appropriately attached to a small molecule which is rapidly cleared from the blood stream. Thus, contrast can be achieved in the images before all of the radioactivity has decayed. On the other hand, a large protein like an antibody is cleared from the blood very slowly and so requires a radionuclide with a much longer half-life (e.g. zirconium-89; t1/2 ~ 3days). That way, radioactivity is still present when image contrast can actually be achieved.
Similarly, the size of the scaffold plays a role in the extent to which modification can be tolerated whilst still maintaining specificity for their target. In short, bigger molecules can tolerate bigger changes. Proteins can often be conjugated to an additional group containing the radionuclide. For small molecules, the radionuclide must usually be incorporated either as a small pendant group or as a substitution for an atom in the naked scaffold.
Frequently, the design of a tracer starts with the modification of a compound which is already known to target a chosen biomarker. In the case of small molecules, approved drugs are sometimes utilised to reduce the burden of optimizing the properties of a compound which allow it to reach its target in the body. That said, producing effective tracers, even from a known starting point, is a difficult task. There is a complex balance of factors to arrive at a tracer that can be made quickly (to maintain the radioactive dose) and augments the properties of the scaffold as little as possible (to ensure effective targeting of the biomarker).
The synthetic routes to radiopharmaceuticals are somewhat different from those familiar to the organic chemist. Short-lived radionuclides such as 18F must be incorporated into the molecule as late on as possible, ideally using high yielding reactions that occur very quickly. This is to ensure that the maximum amount of radiation is available when the tracer is injected into a subject. Consequently, it would be expected that relatively harsh, forcing conditions must be used on highly functionalised molecules which can cause side reactions of the functional groups.
To add an additional challenge into the mix, it is unsafe for chemists to work in close proximity to large amounts of radioactive isotopes. In many labs dealing with radioactive reagents – generally termed ‘hot-labs’ - radiochemists must wear lead aprons and work behind a radiation shield, both of which can be very cumbersome. In the most modern labs and those working with large quantities of tracers, robots are programmed to perform reactions remotely in shielded radiation containment chambers, or ‘hot-cells’.
The synthesis of tracers is teeming with innovation, in both the reactions used to install radioisotopes in or on highly functionalised molecules and also the robots used to perform these reactions. Even when a compound with an established total synthesis is used as the basis of a scaffold, the synthetic route to a related radiotracer may require complete redesign.
With the possibility for customisation to myriad clinical scenarios, nuclear medicine has a bright future. One of the most exciting emerging areas is that of theranostics. In this approach cancerous tumours are first diagnosed using a tracer suitable for a PET scan. Subsequently, the the same scaffold can be used, but the PET suitable radionuclide is replaced with an α-emmitting isotope. The α-emmitting tracer will localise at the same cancerous lesions in the body, causing damage to and killing the cells near the point of localisation due to the highly ionising α-particles, therefore providing a targeted dose of radiation therapy. Within theranostics, technicium-99m and iodine-131 are already used in the diagnosis and treatment of thyroid cancer.
Beyond the chemistry, developments in scanners are also paving the way to higher diagnostic power. Larger fields of view and faster scanning are advantageous for both sensitivity and increasing the amount of information that can be derived from single scans.
Dr Tim Witney’s group at King’s College London are working on the development of tracers which image the ways in which tumour cells resist treatment. Such diagnostic tests would help to stratify relapsed patients to give the most appropriate second-line therapies.
Dr Witney says:
“Nuclear medicine is currently undergoing a revolution. Today, the unique tumour-targeting properties of theranostic agents are being exploited by big-pharma. Following some headline acquisitions in recent months, PSMA-PET imaging is now set to become the new gold-standard imaging technology for metastatic prostate cancer. Outside the applications of nuclear medicine to oncology, Alzheimer’s imaging is providing hope that novel therapies can be actively monitored in real-time. These clinical game-changers are supported by a wealth of basic research, both in the physical sciences in order to make even more complex tracers, and their use by biologists to understand the inner workings of the cell itself.”
With all that’s in the pipeline, we can be sure that this hot topic is not at risk of burning out.
Joseph is a patent attorney working in the chemistry and materials field assisting in the drafting and prosecution of UK and European patents. He also has experience in opposition and appeal proceedings before the EPO and the management of national/regional phase entry of international patent applications.
Email: joseph.newcombe@mewburn.com
Our IP specialists work at all stage of the IP life cycle and provide strategic advice about patent, trade mark and registered designs, as well as any IP-related disputes and legal and commercial requirements.
Our peopleWe have an easily-accessible office in central London, as well as a number of regional offices throughout the UK and an office in Munich, Germany. We’d love to hear from you, so please get in touch.
Get in touch