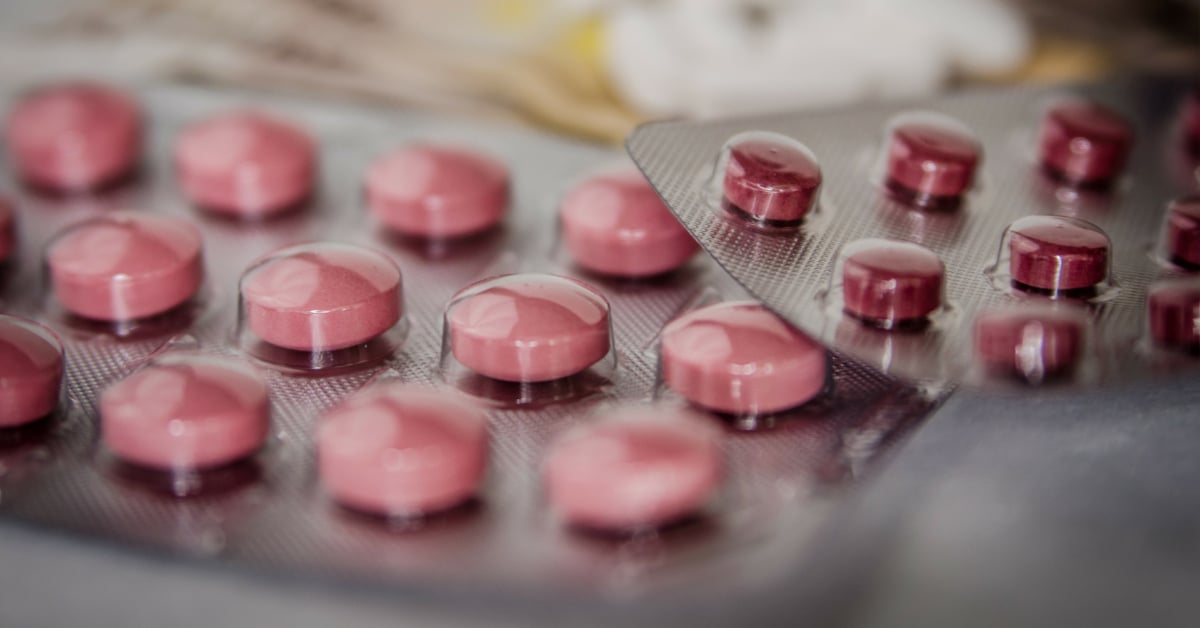
Besides being found within the nucleus and mitochondria of cells, DNA is also present in circulation in the blood plasma. The presence of this circulating free DNA (cfDNA) in the plasma is proving to be of increasing interest for the diagnosis and detection of disease because it offers the opportunity to obtain informative samples from patients without the need for the invasive sampling techniques currently used to obtain tissue biopsies for testing. To distinguish them from conventional tissue biopsies, plasma samples for use in diagnostic and prognostic testing are commonly referred to as “liquid biopsies”.
Liquid biopsies are proving to be particularly useful in oncology. In cancer patients, some of the circulating free DNA that is present in the plasma originates from tumour cells. This subset of the circulating free DNA is known as circulating tumour DNA or ctDNA. ctDNA is very similar in size and composition to circulating free DNA from other sources. However, tumours by their nature contain mutations in their DNA and the presence of these cancer mutations can be used to distinguish ctDNA that originates from tumours from other circulating free DNA. The ability to detect ctDNA in the plasma and distinguish it from other forms of circulating free DNA is important because it is informative about the state of the tumour in the patient and can be used as means to monitor disease burden, response to treatment, and risk of relapse [1],[2].
However, detecting ctDNA in liquid biopsies is technically challenging because the levels of ctDNA having any given cancer mutation may be very low in the plasma of a cancer patient, especially after treatment or surgery. The statistics of sampling mean in any individual sample of plasma from a patient, there may be less than one detectable copy of the ctDNA with the cancer mutation. This may result in the ctDNA not being detected in the patient sample, even though it is in fact present in the plasma as a whole, albeit at low level. This leads to false-negative results in which ctDNA is not detected even though it is present and impacts on the informative value of the liquid biopsy tests for cancer. In some cases, false negatives may mean that the recurrence of a tumour is not detected at an early stage [1],[3],[4].
As well as sampling errors, the sensitivity of the detection of cancer mutations in ctDNA is also limited by sequencing background noise. This occurs when the sequencing signal from the circulating free DNA at the site of the cancer mutation does not reach the threshold required to conclude that the cancer mutation is present, and so ctDNA cannot be detected amongst the other circulating free DNA in the sample.
One approach for improving the sensitivity of liquid biopsy tests for cancer is to increase the number of cancer mutations that are analysed in the sample. Typically, a panel of different cancer mutations is analysed. The presence of any one of the cancer mutations in the panel is sufficient to identify a circulating free DNA molecule as ctDNA, so the level of potentially detectable ctDNA in the sample is increased and hence the sensitivity of the assay improves. The choice of the cancer mutations in the panel may have a significant bearing on the effectiveness of the assay, and the panels of mutations currently used are typically either standardised panels based on known cancer mutations [5], or bespoke panels that are tailored to the specific cancer mutations that are present in the tumour of the individual patient [5],[6],[7]. Although panels of up to 100 cancer mutations have been reported, typical panels contain about 20 patient-specific cancer mutations.
However, even using a panel of cancer mutations may not increase the sensitivity sufficiently to allow reliable detection of ctDNA in plasma samples. For example, despite targeting a panel of about 20 patient-specific cancer mutations, a recent study detected ctDNA in <50% of patients with early-stage NSCLC and failed to detect ctDNA immediately post-surgery in a majority of the patients who later relapsed [6].
A recent paper [8] reports a possible solution to the problem of improving the sensitivity and accuracy of ctDNA detection in liquid biopsies, which may promote the adoption of liquid biopsies in the clinic for monitoring cancer patients more widely. This solution relies on next-generation sequencing (NGS) technology both to identify large numbers of the cancer mutations that are present in an individual patient's tumor and to sequence large amounts of circulating free DNA in a sample of plasma from the patient. ctDNA sequences containing one or more of the cancer mutations are then detected amongst the sequences of circulating free DNA using bioinformatics. In particular, an algorithm (known as INtegration of VAriant Reads (INVAR)) is used to generate a signal that represents an aggregate of the hundreds or thousands of the sites where cancer mutations may occur in the patient. The aggregation of signal from this many sites is technically challenging and requires the use of number of different error suppression and signal enrichment steps. The aggregate signal is then compared to the background. A signal that is significantly above background indicates the presence of ctDNA in the sample.
Using this approach, residual ctDNA was detected down to levels of individual parts per million and below, an improvement of up to an order of magnitude over existing techniques. For example, ctDNA was detected in 100% of patients with stage IV breast cancer or melanoma and 62.5% of patients with glioblastoma. In earlier-stage disease, ctDNA was detected in 63% of patients with lung cancer before treatment, in 29% of patients with early-stage melanoma after surgical resection (40% of those who later relapsed) [8].
These improvements in the sensitivity are likely to encourage the adoption of liquid biopsy techniques across oncology. As their advantages are recognised, the commercial value of these techniques will grow and IP protection will become an increasingly important issue for businesses seeking to develop assets in this field. The complex combinations of biology and information processing that lie at the heart of these techniques will present challenges to patent practitioners and a considered and forward-looking IP strategy will be important in protecting these assets.
Nick is a Partner, Patent Attorney and Litigator at Mewburn Ellis. He works across the full range of patent activity in the life sciences sector, from pre-drafting advice and drafting of applications to worldwide portfolio management, prosecution and appeal. Nick is also experienced in defensive and offensive European oppositions and due diligence work.
Email: nick.sutcliffe@mewburn.com
Our IP specialists work at all stage of the IP life cycle and provide strategic advice about patent, trade mark and registered designs, as well as any IP-related disputes and legal and commercial requirements.
Our peopleWe have an easily-accessible office in central London, as well as a number of regional offices throughout the UK and an office in Munich, Germany. We’d love to hear from you, so please get in touch.
Get in touch