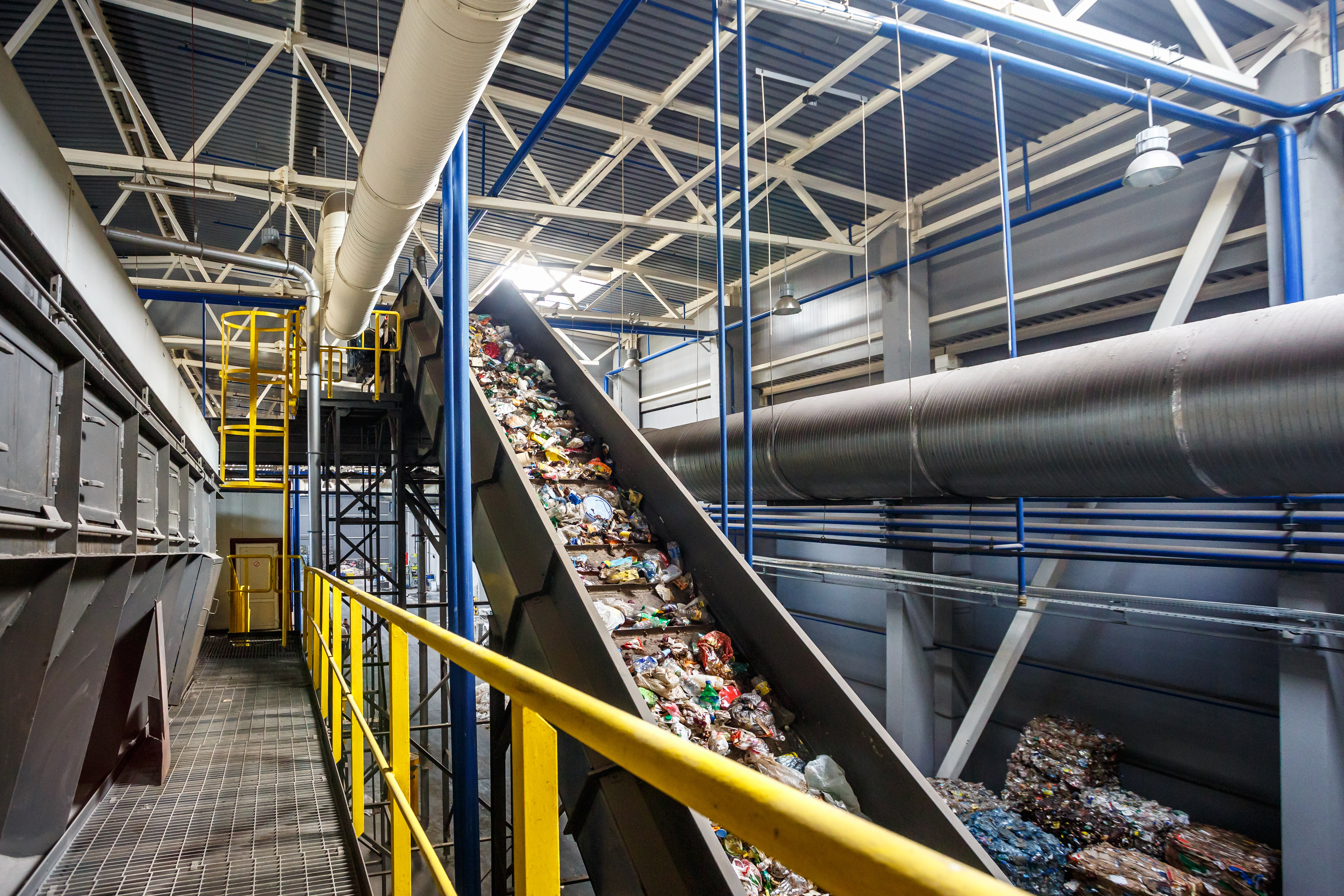
Will the world's most dangerous microbes soon meet their match? Forward finds out what's in the pipeline when it comes to stopping superbugs.
Forward: features are independent pieces written for Mewburn Ellis discussing and celebrating the best of innovation and exploration from the scientific and entrepreneurial worlds.
Its an issue of global magnitude. Its approach has been foretold for decades. Its advance threatens the lives of millions of people. It will require exponentially more funding and public awareness in order to stop its progress.
If you thought the threat being described was climate change, that would probably be because that crisis has rapidly gained a space in the public conversation over the pest year. But these statements actually set out the threat posed by antimicrobial resistance (AMR).
AMR arises when microbes such as bacteria learn to evade the mechanisms of the drugs used to attack them. Antimicrobial drugs are becoming ineffective against commonly acquired bacterial infections at an ever increasing rate. In some cases the drug-resistant bacterial strains are emerging within only a few years of the drugs first being prescribed. This has serious implications for the risks associated with surgery, minor injuries and hospital stays. Put simply, AMR is a threat which affects us all
Each year, as many as 700,000 people worldwide die due to treatment-resistant infections. Projections suggest that this figure may rise to ten million per year by 2050 unless new and innovative solutions are found.
The main offenders are well known. A set of six pathogens - known collectively as ESKAPE to reflect their first initials - bears primary responsibility for diseases acquired in a hospital setting (so-called nosocomial infections). These pose a particular problem for the modern healthcare system, as infections can spread rapidly through vulnerable patient populations.
In addition to the ESKAPE pathogens, the US Centers for Disease Control and Prevention (CDC) also includes Candida auris, Clostridioides difficile and the drug-resistant strain of Neisseria gonorrhoeae on a list of 'critical threats'.
The CDC is one of the many agencies warning that we have entered a 'post-antibiotic' era. Quite simply, we cannot continue to use and rely on antibiotics as we have in the past. As such, this requires us all to be responsible in our use of antibiotics and to work to prevent the spread of infection. Widespread reliance on antibiotics helps microbes to learn and evolve, developing resistance to those drugs.
Among the simple precautions recommended by the World Health Organization (WHO) to slow the progress of AMR are: practising good hand hygiene in medical settings; vaccination programmes; and the use of barrier methods to prevent the spread of sexually transmitted infections. The message from the WHO is that 'the future of antibiotics depends on all of us'.
But perhaps the future relies on some people more than others, particularly those offering new research and thought leadership on this subject. With this in mind, Forward set out to identify some of those at the forefront of the fight against AMR.
For Professor Steffanie Strathdee, co-director of the Center for Innovative Phage Applications and Therapeutics (IPATH) at the University of California, San Diego, the fight against AMR is distinctly personal. Her husband was treated intravenously with phage therapy as a last-ditch attempt to rid him of Acinetobacter baumannii after all other treatments had failed. Long story short: the treatment worked, and Strathdee’s account of what happened (as told in her book The Perfect Predator) has galvanised a new phase of acceptance for and research into phage therapy, which she describes as ‘a 100-year-old and largely forgotten cure’.
As she explains, phages (short for bacteriophages) are viruses that have naturally evolved to attack bacteria. They are very specific, so an individual phage will only kill the bacteria that it is a match to. Once introduced to the patient, the live phage attaches to a bacterial cell through a receptor, injects its genetic material into the cell and turns it into a phage manufacturing plant. On a kill signal, up to 300 ‘baby phages’ burst out of the bacterial cell and destroy it – along with the rest of the bacteria that they match. Finally, they are naturally excreted by the body with no harm to other beneficial bacteria or to human tissues.
Phage therapy isn’t currently licensed for use in the US, so there are a number of hurdles still to overcome. But these are not insurmountable, says Strathdee. She envisions the creation of an open-source phage bank holding thousands of phages that have been characterised and sequenced so that users know what receptors they have and which phages work well with each other. ‘This is important, because you really need a phage cocktail to attack the bacteria to avoid resistance. My husband’s infection was caused by one of the ESKAPE pathogens. You might need 300 or so phages to cover the majority of the circulating strains of the bacteria he had.’ This should not be unachievable: Strathdee notes that there are 1031 phages on our planet. For context, that makes the bacteriophage population larger than the populations of all other organisms, including bacteria, put together.
At present, each phage treatment is personalised, but Strathdee believes it may be possible to develop a range of phage cocktails that could be sold and used where it is shown that an infection is a match for a particular group of phages. Natural (also known as wild-type) phages can be genetically modified so they attack a larger number of strains, and there are companies currently working on producing new phages via synthetic biology.
IPATH, the first dedicated phage therapy centre in North America, will soon be enrolling patients with cystic fibrosis for a clinical trial funded by the National Institutes of Health (NIH) as part of the Antibacterial Resistance Leadership Group. If it goes well, several other institutions in that network will enrol patients as well. ‘That is very exciting as it is the first time the NIH has invested in phage therapy like this,’ Strathdee says. IPATH is also doing translational research to determine the best doses and routes of administration.
For the first time, NIH has issued a request for proposals on basic and clinical research to support phage therapy trials. And the Mayo Clinic carried out its first phage therapy treatment last summer on a patient who had undergone 17 operations and had just picked out a wheelchair having been told that an amputation would be necessary. After one or two infusions, his infection cleared. The Clinic is now treating other patients in a similar way.
Strathdee and her colleagues view phage therapy as complementary to, not a replacement for, antibiotics. ‘The selective pressure that phages and antibiotics put on bacteria can make it mutate and suffer a genetic penalty in doing so, making it susceptible to the antibiotic again. We need to study this more so that we can take advantage of that synergy,’ she says. Ultimately, Strathdee tells Forward: ‘I am glad a conversation has been opened.’
The work being done by Procarta Biosystems is ‘technically complex’, but Dr Martin Stocks, chief operating officer, offers Forward the simplest explanation he can. Stocks refers to the core technology as ‘precision antibiotics’. In other words, Procarta is aiming to create drugs that kill specific harmful bacteria but preserve the beneficial bacteria that the body needs.
‘Our lead antibiotic is semi-precision, so it kills a large group of bacteria rather than one specific type. We’re going after the CREs [Carbapenem-resistant enterobacteriaceae, see page 27], often referred to as opportunistic pathogens. If they get into the wrong bit of the body, say the urinary tract or a perforated bowel, they can cause very unpleasant diseases. They are particularly adept at acquiring resistance to antibiotics, both in terms of creating mutants but also in that if one acquires resistance, that very rapidly spreads throughout the population.’
Uniquely, Procarta is developing a technology that will allow it to produce many different antibiotics rapidly. Stocks explains: ‘We are targeting the fundamental control systems that make the targets and everything else in the bacteria – the transcription process. The beauty of it is that the bacteria cannot mutate the control system without effectively committing suicide. Transcription factors are the machinery that turns genes on and off, and they fundamentally control how the entire physiology of the bacteria operates. If a transcription factor is mutated, it will affect hundreds if not thousands of genes and the way they are expressed, so it is very difficult for the bacteria to protect themselves against our agents.’
Procarta recently received a funding award from Combating Antibiotic-Resistant Bacteria Biopharmaceutical Accelerator (CARB-X). This, says Stocks, ‘is absolutely critical for the stage we are at’. ‘It will take Procarta’s technology to Phase 1 clinical trials, about two and a half to three years from now. Realistically, though, it will be seven to ten years before a solution reaches the market’.
While Stocks says he would never downplay the challenges in reaching a therapeutic outcome, he is very optimistic. ‘We have a long way to go, but I think the tech is very powerful and that we will be able to deliver something of value.
‘There is nobody globally doing anything like what we are doing. We are either an innovator or completely barking; that is the balance we strike. Hopefully, we will prove to be the former and not the latter.’
For such simple and seemingly primitive organisms, bacteria are surprisingly sophisticated in their interactions with each other. And the tools that bacteria use to fight each other may hold the key to a new antibiotic pipeline, research from the MRC Centre for Molecular Bacteriology and Infection (CMBI) at Imperial College London suggests.
Professor Alain Filloux tells Forward how exploiting a tool used by the bacteria Pseudomonas aeruginosa to attack rival bacteria – a tool referred to as a ‘toxic arrowhead’– might enable the development of a mechanism to compromise the structural integrity of resistant bacteria cells.
The magic ingredient in this effort is VgrG2b. This toxin, according to Imperial College, ‘targets the cell envelope between the inner and outer bacterial membranes and encompasses the cell wall… [It] prevents the cell from dividing, causing it instead to bulge and eventually explode as the integrity of the cell fails’.
This is just one of the mechanisms that Filloux says could potentially be mimicked to develop antimicrobials. In short, bacteria have a number of toxins that they inject directly into each other, and the key thing is finding the targets of those. ‘These targets have never been exploited in terms of antimicrobials, so you can replenish the pipeline of antibiotics by implementing new drugs against new targets,’ he explains.
The researchers have been working on this area for ten years and have achieved proof of concept, he says, but it is by no means a quick fix. There is a huge distance between the academic work and the industrial/pharmaceutical end of the process. While the support of pharmaceutical companies and industrial partners might make it possible to identify particular molecules within the next five years or so, no one can predict how long it will take to get any of those molecules to the market. ‘That is the whole problem of an antimicrobial pipeline which is actually draining. We can pinpoint the targets, but we need greater support and funding to get further,’ says Filloux.
‘We would like to see an AMR centre dedicated to the study of bacterial pathogens and basic research, and visible to the public, government bodies and pharma companies so we can really start to generate momentum to get those molecules into the pipeline.’
Evidence tells us that overexposure to antibiotics helps microbes improve their defences: they learn and evolve through these encounters. Using the right drug at the right time is crucial in this fight.
This is the focus of the Longitude Prize, which will award £8m to the first researchers to develop an affordable and rapid (within 30 minutes) diagnostic test for bacterial infections that can be used easily, anywhere and in any health setting.
The need for such a test is urgent: it will enable health service providers to avoid prescribing precautionary, incorrect or broad-spectrum treatments that are some of the drivers for the spread of antibiotic resistance. For example, more than 90% of patients seeking treatment for an acute cough are suffering from a virus which antibiotics cannot treat.
Some 57 teams have already thrown their hat into the ring. Although many are said to have made significant progress, the deadline for the prize has been extended and the competition will be open until at least December 2020. So far, the judging panel has reviewed the work of 14 teams, none of which met all of the necessary criteria, so the extension, according to the organisers, ‘will not only give the teams more time to work on their diagnostic technologies, it also gives them more time to find additional funding and establish new collaborations with partners’.
Launching the competition in 2014, Geoff Mulgan, chief executive of the National Endowment for Science, Technology and the Arts, set out the ultimate goal: ‘Our hope is that the combination of the prize and greater awareness of the problem will fuel a dramatic acceleration in the search for solutions, many of which will be surprising and from unexpected sources.’
This is a fight we must winSarah Kostiuk-Smith, a member of the chemistry and materials patent team at Mewburn Ellis, says:
|
A who's who of the bacteria causing the most concern — the ESKAPE group
Enterococcus faecium
Causes endocarditis and urinary tract, wound and bloodstream infections. It has become increasingly resistant to a range of commonly used antibiotics.
Staphylococcus aureus
Said to be present in a third of all humans, often without becoming symptomatic, it can cause skin and soft tissue infections. Strains of S. aureus are now resistant to antibiotics like methicillin. These variants are known as MRSA.
Klebsiella pneumoniae
A scourge of the world’s intensive care units, it often preys on patients reliant on ventilators or catheters. The genus is responsible for a wide range of diseases but the most common condition is pneumonia.
Acinetobacter baumannii
This opportunistic pathogen can cause pneumonia, meningitis and wound, bloodstream and urinary tract infections, posing a particular threat to hospital patients.
Pseudomonas aeruginosa
This bacteria often attacks those with underlying conditions such as cystic fibrosis, but also causes pneumonia and infections of the blood.
Enterobacter
Technically named Enterobacteriaceae, members of this large family of bacteria cause respiratory and urinary tract infections. The rise of highly resistant bacteria within this family is of increasing concern. (See CREs below.)
Candida auris
This multidrug-resistant yeast causes infection and is easily spread. First identified in 2009, some types are resistant to all available classes of antifungals. Four different strains have emerged at the same time across the globe.
Clostridioides (clostridium) difficile
Though it is rarely resistant to antibiotics itself, it often attacks those taking antibiotics for another condition and can cause life threatening
Neisseria gonorrhoeae
Causes gonorrhoea, which is sexually transmitted and has developed resistance to all but a single class of antibiotics. It is often symptomless, so people may not be aware they are infected until serious health problems occur.
Carbapenem-resistant enterobacteriaceae (cres)
CREs are resistant to many antibiotics, including carbapenems – often claimed to be ‘the last line of antibiotic defense’ against resistant pathogens. These strains are resistant because they produce an enzyme called a carbapenemase, which disables the carbapenem drug molecule. Resistance varies from moderate to severe.
This article was originally published in the third edition of Forward Magazine. You can view it here.
Julie specialises in the drafting and prosecution of patent applications with a particular emphasis on both organic and pharmaceutical chemistry. She has extensive experience of advising on infringement and validity issues, in particular providing Freedom-to-Operate searches and opinions. She also represents clients in EPO opposition proceedings and provides general advice to intellectual property, including international portfolio management, IP strategy and IP litigation.
Email: julie.carlisle@mewburn.com
Our IP specialists work at all stage of the IP life cycle and provide strategic advice about patent, trade mark and registered designs, as well as any IP-related disputes and legal and commercial requirements.
Our peopleWe have an easily-accessible office in central London, as well as a number of regional offices throughout the UK and an office in Munich, Germany. We’d love to hear from you, so please get in touch.
Get in touch