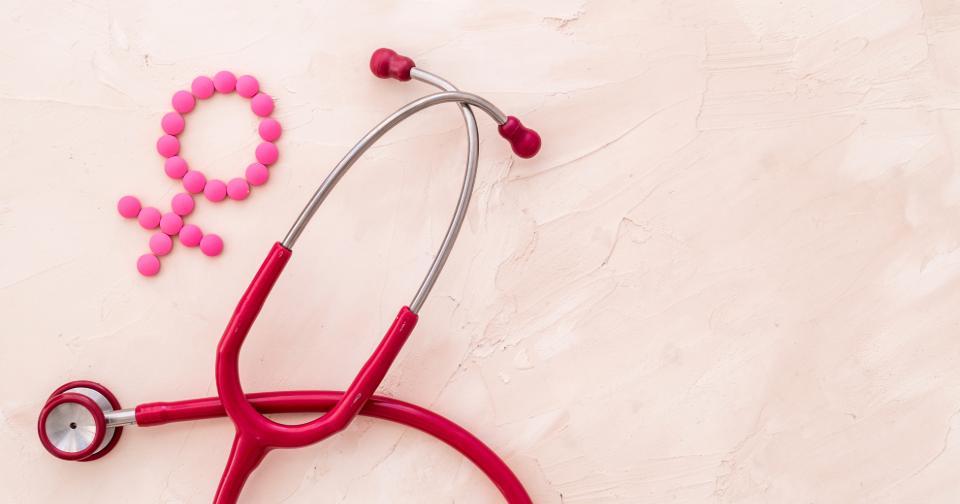
Recent cell and gene therapy product approvals have allowed the pharmaceutical industry to start a new age of innovation regarding cancer treatment, moving away from the more homogeneous therapies of the past.
The past decade has seen unprecedented advances in cancer treatment, shifting from a ‘one-size-fits-all’ approach towards the clinical development of technologies that engage a patient’s own immune system to fight off cancer cells. These cancer immunotherapies rely on the ability of the host’s immune system to recognise tumour antigens and effectively eradicate tumour cells.
Chimeric antigen receptor (CAR) T cell therapy, has gained lots of attention in recent years with the landmark approvals of the first two CAR T cell therapies, KymriahTM and YescartaTM, first by the FDA in 2017, with the European Medicines Agency quickly following suit in 2018. These first approvals are only the start of this new era in cancer medicine, with the FDA predicting that it will approve 10-20 cell and gene therapy products a year by 2025[1]. While there were concerns initially that current health systems might not be supportive due to the high costs associated with these one-off treatments, the manufacturers, Novartis (Kymriah) and Gilead (Yescarta), managed to successfully obtain reimbursement in many countries in Europe[2]. It signals that governments and healthcare systems are keen to implement these innovative treatments, which, in turn, provides impetus for companies to invest in these treatment areas.
CAR T cell therapy uses the patients’ own modified T cells to recognise antigens expressed by cancer cells and subsequently kill those cells. However, in many cases, these antigens are also expressed by normal, non cancerous cells and tissues – it’s just that they’re overexpressed or misregulated in the cancer cells. As these so-called ‘common’ antigens are not tumour-specific, one factor limiting the clinical application of CAR T cells are severe toxic side effects, such as cytokine release storm and neurological events. There are ongoing developments designed to minimise these side effects associated with CAR T cell therapy.
One promising development is the adoptive cell transfer (ACT) of T cells specific for neoantigens[2]. These neoantigens are peptides generated by somatic mutations within cancer cells, and as such are absent from normal tissues and cells. As opposed to the common antigens targeted by CAR T cells, neoantigens are mostly tumour- and patient-specific. The likelihood of the severe toxic off-target effects resulting in damage and destruction of normal cells and tissues is, therefore, limited. Neoantigens offer an exciting new option for immunotherapy.
However, neoantigens and T cells specific for these antigens are typically present at very low frequencies, which makes identifying them challenging. Advances in deep sequencing technologies and in silico prediction algorithms have allowed rapid identification of candidate neo-epitopes[3]. Moreover, innovative methods for isolating neoantigen-specific T cells ,from tumour tissue and blood have been developed, such as nanoparticle-barcoded nucleic acid cell sorting, which uses fluorescent DNA barcodes presented on nanoparticle scaffolds for the detection and isolation of neoantigen-specific T cells[4]. After isolation, the neoantigen-specific T cells are expanded to obtain sufficient numbers of effector T cells for infusion back into the patient. Alternative methods for generating populations of neoantigen-specific T cells for use in the treatment of cancers by adoptive transfer include cloning T cell receptor genes from neoantigen-specific T cells for expression in a distinct population of effector T cells.
While clinical studies using ACT of neoantigen-specific T cells have produced promising results, several challenges remain[5]. For example, neoantigen-specific T cells currently need to be expanded ex vivo for several weeks before they can be reintroduced in the patient. These time scales are simply incompatible with rapidly progressing cancers. The speed of producing the populations of neoantigen-specific T cells needed will, therefore, need to be increased to meet the therapeutic demand for the treatment of a wide range of cancers.
Cancer cells have several mechanisms in place to prevent detection and attack by the immune system. These mechanisms include immunoediting, which promotes the loss of neoantigens recognised by the T cells during tumour development. It may, therefore, be necessary to devise strategies to minimise or prevent tumour immune evasion by further editing the mutated antigen when designing these therapies[6].
Complex treatments also require highly specialised laboratories for the production, selection, and storage of neoantigen-specific T cells, as well as the technical capability for the extraction and reintroduction of the re engineered T cells. This sort of infrastructure is not widely available around the world, posing logistic and financial barriers for patients wanting to access this type of treatment.
Due to the cost associated with ACT, these therapies will probably be largely focused on rarer types of cancers. With relatively low patient numbers suffering from such diseases, clinical trials will be more complex and will likely take place across multiple countries.
Lastly, while the recent reimbursement deals in many European countries indicate that governments are supportive of these novel technologies, the hefty price tag of up to $500,000 per patient remains a barrier for current subsidised and national healthcare systems to fund these treatments. Outcome-based payment schemes, where a medicine’s price is linked to patient outcomes, have become increasingly common in Europe and the rest of the world[7][8]. The successful implementation of these outcome-based payment models will largely depend on well-designed policies, data collection and real-word evidence systems, and agreements between the companies and health care systems[9].
Oncology is one of the world’s fastest growing treatment areas and the global spending on therapeutic cancer drugs is expected to exceed $200 billion by 2023, with an average growth of up to 14%[10]. The success of treatments such as Kymriah has spurred investment, innovation, and growth in the field of adoptive T cell transfers and the global adoptive T cell market alone has been predicted to expand to $8 billion by 2028[11].
Given the high profits anticipated by these therapies, it is perhaps not surprising that innovators have been striving to protect their market share by filing patents covering many aspects of their inventions.
With such high stakes, it is also unsurprising that we’re already seeing litigation in this space. Indeed, attempts at patent litigation between CAR T cell companies began even before the therapy had been approved, with Juno Therapeutics (now part of Bristol-Myers Squibb) suing Kite Pharma (a subsidiary of Gilead) for infringing a CAR T cell technology invented and patented by Sloan Kettering and licensed exclusively to Juno. In a historical verdict last year, a US jury ordered Kite Pharma to pay nearly $800 million to Juno therapeutics for infringement.
Innovators should therefore be taking steps to ensure that they have freedom to operate at an early stage in R&D and clinical development. By identifying patent rights that could be problematic early on, it is possible to take preventative action. This could be done by making technical changes to the engineered T cells, adjusting production protocols to try to work around other people’s IP rights. Alternatively, steps could be taken to try prevent a patent being granted, or to challenge a granted patent, to try to clear the way.
Developing an effective patent strategy for these novel neoantigen-based technologies is not as straightforward as those for the classic ‘off-the-shelf’ pharmaceutical products, where the substance or drug is well defined and can be mass produced. As each patient receives their own unique therapeutic T cell batch, the actual product for these new adoptive T cell technologies is different for each patient. Enforcement of product (composition of matter) claims relating may, therefore, be challenging, where each batch of T cells must be demonstrated to fall within the patent claims. This is particularly the case for neoantigen targeting therapies, where the T cells may be very different for each patient, having different T cell receptors targeting different antigens.
On the other hand, claims directed to methods for isolating, engineering, and expanding neoantigen-specific T cells, as well as methods for identifying neoantigens, can be of real value. In contrast to existing CAR T cell therapies, where T cells recognise a common target such as CD19, ACT of neoantigen–specific T cells is even more precise and targets will be different between patients as well. This means that a process of neoantigen identification and T cell production may be needed for each patient.
Granted claims around approved methods could be more commercially useful and enforceable as regulatory agencies will probably want any party wishing to bring therapies to the market to disclose the exact methods they will use to engineer their T cells.
However, care must be taken to ensure that the resultant claims are effective deterrents and can be enforced against a potential infringement. With different parties undertaking different steps of a method, careful patent drafting is required to avoid allegations of divided infringement, where no single party is responsible for undertaking all the steps of a patented method, thereby avoiding infringement. Indeed, for ACT using neoantigen-specific T cells, the rare nature of the antigens and cancers involved, it’s likely that different parts of the method will take place in different jurisdictions. For example, a patient’s T cells may be extracted in one country, and genetically modified in another, and thus, the method as a whole is not performed in any one country. A globally coordinated strategy for obtaining, enforcing, and defending patent rights will therefore be essential for these types of technologies, because of the geographically limited nature of IP rights.
Finally, we note that while the US remains the biggest market in the field of oncology, China’s share has grown significantly in the last few years. With China emerging as one of the major players in the global oncology market, it will be worth keeping the Chinese patent system in mind when drafting applications and obtaining patent rights[12]. For example, the Chinese patent office usually requires the claims to closely match the experimental data in the application in order to grant the patent. Including supporting data in the application at the outset is therefore critical to success in obtaining patents in China.
ACT of neoantigen-specific T cells has emerged as a promising new type of cancer immunotherapy. While investment and research in the field are growing, several challenges remain, meaning there is huge scope for innovation. With the adoptive T cell market not only being lucrative but also highly competitive, innovators should consider securing their IP rights and considering the impact of other parties’ IP carefully, and early, in development of these therapies.
1. Visit: www.fda.gov/news-events/press-announcements/statementfda-commissioner-scott-gottlieb-md-and-peter-marks-md-phddirector-center-biologics
2. Jørgensen J et al, Outcomes-based reimbursement for gene therapies in practice: the experience of recently launched CAR-T cell therapies in major European countries, J Mark Access Health Policy 8(1): 2020
3. Visit: science.sciencemag.org/content/348/6230/69.long
4. Arnaud M et al, Biotechnologies to tackle the challenge of neoantigen identification, Curr Opin Biotechnol 65: pp52-9, 2020
5. Peng S et al, Sensitive detection and analysis of neoantigenspecific T cell populations from tumors and blood, Cell Rep 28(10): pp2,728-38, 2019
6. Jiang T et al, Tumor neoantigens: From basic research to clinical applications, J Hematol Oncol 12(1): p93, 2019
7. Schreiber RD et al, Cancer immunoediting: integrating immunity’s roles in cancer suppression and promotion, Science 331(6024): pp1,565-70, 2011
8. Visit: heatinformatics.com/posts/outcome-based-payment
9. Visit: pharmaboardroom.com/articles/shooting-for-thestars-the-evolution-in-outcome-based-payment-models-formedicines
10. Visit: www.iqvia.com/insights/the-iqvia-institute/reports/global-oncology-trends-2019
11. Visit: www.coherentmarketinsights.com/press-release/globalcar-t-cell-therapy-market-to-reach-us-77-billion-by-2028-18
12. Visit: http://ipimediaworld.com/wp-content/uploads/2018/01/The-rise-of-cellular-immunotherapy.pdf
This article is taken from European Biopharmaceutical Review October 2020, pages 53-55. © Samedan Ltd
You can navigate to the original article from the contents page of this issue here.
This article was co-authored by Fran Salisbury and Ine Vanderleyden.
Ine Vanderleyden is a trainee patent attorney and member of the life sciences patent team at Mewburn Ellis. She has a BSc and MSc in Biomedical Sciences from KU Leuven, Belgium, and a PhD from the University of Cambridge. Her doctoral research focussed on the role of regulatory T cells in controlling immune responses, with a focus on the germinal centre response. Ine's subsequent postdoctoral research investigated the transcriptional regulation of regulatory T cell development.
Fran is a Partner and Patent Attorney at Mewburn Ellis. She works in all patent cycle stages within the life sciences sector – from invention capture, drafting and patent strategy to prosecution and global portfolio management. Fran is a member of our plant variety rights team, having completed the official WIPO course on plant variety protection under the UPOV convention. As such, she has extensive experience dealing with plant-related inventions, including obtaining plant variety rights and entry onto the national list and common catalogue.
Our IP specialists work at all stage of the IP life cycle and provide strategic advice about patent, trade mark and registered designs, as well as any IP-related disputes and legal and commercial requirements.
Our peopleWe have an easily-accessible office in central London, as well as a number of regional offices throughout the UK and an office in Munich, Germany. We’d love to hear from you, so please get in touch.
Get in touch