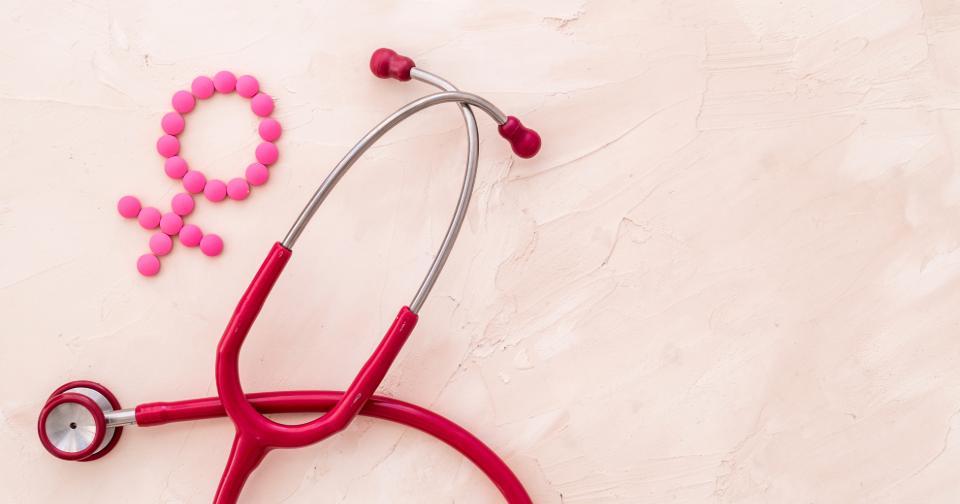
Recent advances in medicine have, for the first time, allowed physicians to directly intervene in the body’s cellular response to disease. New cell-based treatments have transformative potential, and the approval in recent years of several medicines in this area offer hope that a new era of medical science is dawning.
Cell therapy – where new, healthy cells are introduced into a patient’s body to replace or augment cells that are diseased or absent – traces its origins back as far as 1939, when the first (unfortunately unsuccessful) bone marrow transplant was attempted for a patient with aplastic anaemia. Success finally came in 1968, and since then bone marrow transplant has become a common treatment for patients with cancers of the blood, such as leukaemia, following destruction of their diseased cells by chemotherapy or radiation. Despite the risks of complications from the invasive procedure, bone marrow transplants have significantly improved the prognosis of leukaemia patients.
Other tissues also lend themselves to cell transplant. For example, cartilage at joints can wear away over time, particularly in the knee, resulting in significantly impaired mobility. Spherox, first offered in the UK in 2018, addresses this through cell transplant: a small cartilage biopsy is obtained from the patient and cultivated to form spherical clusters of cartilage-generating cells called chondrocytes, which are transplanted into the knee to regenerate damaged cartilage. The “autologous” nature of the cells – the fact that they are obtained from the patient in question – removes the need for complex tissue type matching and the risks of transplant rejection inherent with donor or “allogeneic” transplants.
A similar approach has also been utilised for treating defects of the skin. The autologous skin cell product Azficel T (Laviv) was approved as long ago as 2011 for treatment of nasolabial fold wrinkles, and its manufacturer (now Castle Creek Biosciences) is developing similar treatments for a range of skin diseases.
A major obstacle for transplantation cell therapy is obtaining enough cells. Furthermore, direct donation is only possible for cell types which are easily accessible, essentially limiting the types of condition that can be treated in this way. Some of these issues can be overcome with stem cells. These are unspecialised cells that, unlike most, can divide, multiply, and develop into other functional cell types. This may be performed outside the body, allowing large quantities of cells to be generated from a small sample.
Two main types of stem cells are being explored in the context of cell therapy: pluripotent stem cells (which can form a large number of cell types) and tissue-specific stem cells (which have a limited number of eventual fates). Pluripotent stem cells can be seen to have wider potential utility than tissue-specific stem cells, but directing their differentiation into specific cell types is technically challenging and the use of human embryonic stem cells is ethically controversial. Tissue-specific stem cells can be isolated from donors (e.g. from bone marrow or umbilical cord) and form the basis of all stem cell therapeutics approved to date. In regenerative medicine, the tissue-specific stem cells of greatest interest are haematopoietic stem cells (HSCs), which give rise to blood cells, and mesenchymal stem cells (MSCs), which give rise to tissues such as muscle, bone and connective tissues.
The world’s first approved stem cell therapy was Remestemcel-L, a suspension of allogeneic bone marrow-derived MSCs used for treatment of steroid-refractory graft-versus-host host disease. Initially approved in Canada in 2012, the product has since been approved in Japan and is under review in the USA. Takeda’s Alofisel (darvadstrocel), a treatment for complex perianal fistulas in sufferers of Crohn’s disease using adipose-derived MSCs, became Europe’s first approved allogeneic stem cell therapy in 2018.
Umbilical cord blood, rich in haematopoietic stem and progenitor cells (HSPCs), is useful in treating a variety of disorders, from blood and bone marrow cancers to sickle cell anaemia. Many new parents now choose to cryopreserve the cord blood (collected after the baby is delivered and the cord is cut), either for donation (whereby it is processed into products such as Allocord and Hemocord) or “banking” as autologous stem cells in case their child should need them in later life.
A particular type of eye stem cell, called a limbal stem cell, is used in Holoclar, a treatment for limbal stem cell deficiency (LSCD), which in 2015 became Europe’s first approved stem cell therapy. This autologous limbal stem cell transplant can allow LSCD sufferers to recover their sight without requiring a corneal transplant.
So far, stem cell-based therapies for regenerative medicine are limited to tissue types with diffuse structures, due to the challenge of recreating the highly ordered tissue microenviroments required for normal development. As technology to create tissue scaffolds develops, stem cell therapies may eventually be used to regenerate large, complex tissues and organs in situ, increasing their clinical usefulness significantly (see our blogs Collagen scaffolds – the future of tissue engineering? and The future of organ transplantation: growing organs from scratch?).
Excitingly, gene therapies using genetically modified stem cells have also now been approved. Libmeldy recently hit the headlines after successfully curing a child from the fatal genetic disorder metachromatic leukodystrophy, which results from a defective arylsulfatase A (ARSA) gene. The therapy uses autologous CD34+ enriched HSPCs transduced with a lentiviral vector carrying a functional ARSA gene. The modified cells take root in the patient’s bone marrow, replicating and creating cells that mature and create normally functioning ARSA. Another therapy utilising modified autologous stem cells is Zynteglo, in which HSPCs are modified to express haemoglobin for the treatment of beta-thalassaemia.
The ability to modify a patient’s cells in this manner could one day provide therapies for countless debilitating genetic diseases. However, the success of this depends on stable, safe, and reliable vectors for the modification of stem cells, as well as mastering their enrichment and expansion. Innovators working in this field may find that their platforms for transformation, culture, and differentiation have widespread applicability, meaning that developers are able to license their technology to groups working on many different diseases. Indeed, given the regulatory network that is arising around cell therapies, methods of ensuring and measuring quality control may prove especially valuable in the long run, especially if these become industry standard.
Rather than cellular transplant, another type of cell therapy is cellular immunotherapy, in which immune cells are modified to endow them with entirely new capabilities not seen in nature. In CAR-T therapy, T cells are modified to express a chimeric antigen receptor (CAR), a synthetic receptor that recognises a target antigen that is found on cancer cells. Expression of a CAR in a T cell turns the T cell into a “living drug”, which hunts down cancer cells and destroys diseased tissues in a patient (see our blog Fighting cancer with CAR-T therapies).
Many CAR-T therapies on the market target CD19, a B-cell marker that is overexpressed on cancers arising from these cells. Approved by the FDA in 2017, Yescarta (Kite Pharma), a treatment for large B-cell lymphoma, and Kymriah (Novartis), approved for use against B-cell acute lymphoblastic leukaemia, have been swiftly followed by Breyanzi (Bristol-Meyers Squibb) and Tecartus (Kite Pharma), the latter of which is now offered under the NHS to mantel cell lymphoma patients. Also now approved are Abecma (Bristol-Meyers Squibb) and Carvykti (Janssen) which target B cell maturation antigen (BCMA) to treat myeloma. These therapies all use autologous T-cells, meaning that the treatments are personalised for each patient, making CAR-T therapy currently an expensive procedure. As CAR-T therapies advance, it may one day be possible to develop allogeneic therapeutics which work “off the shelf” for a multitude of patients, reducing costs and increasing availability, or to use T cell engagers to direct unmodified T cells to a tumour in vivo.
Recent years have seen significant advances in cell therapy, and after bone marrow transplant, approval and commercialisation of the first cellular therapeutics. As cell therapies continue to develop, they have the potential to provide new treatments for myriad diseases. We are excited to see the continued progress of the field in the years to come.
Ed handles patent work across the life sciences/biotech field, with a particular focus on antibody therapeutics, cell therapy and vaccines. Outside of the medical sphere he also has extensive experience in enzyme technology. Ed is very experienced in drafting patent applications and prosecuting them around the world, and also handles opposition and appeal work at the European Patent Office.
Email: edward.couchman@mewburn.com
Our IP specialists work at all stage of the IP life cycle and provide strategic advice about patent, trade mark and registered designs, as well as any IP-related disputes and legal and commercial requirements.
Our peopleWe have an easily-accessible office in central London, as well as a number of regional offices throughout the UK and an office in Munich, Germany. We’d love to hear from you, so please get in touch.
Get in touch