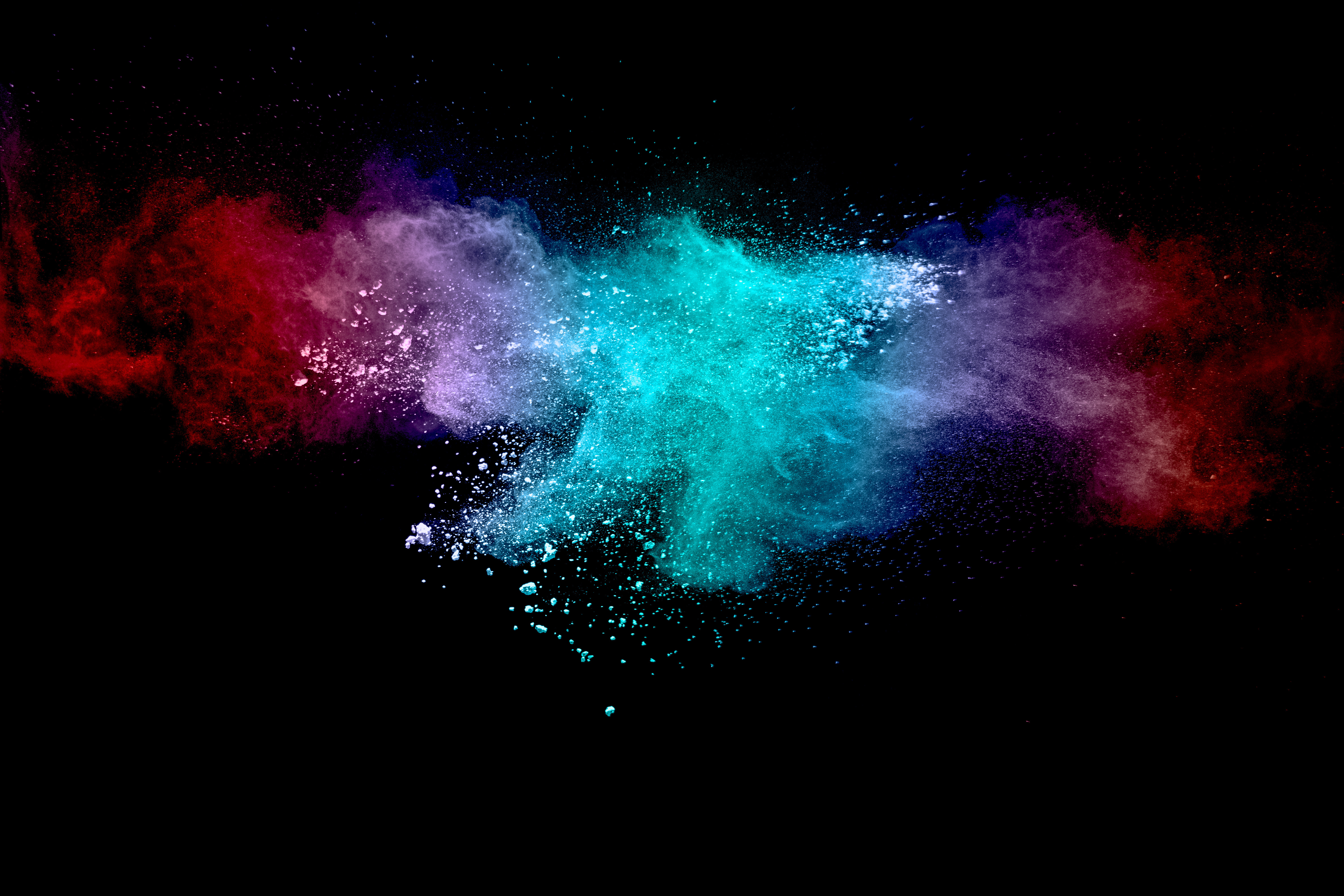
As the UK begins work towards realising world-beating ambitions, Forward set out to learn just what is so compelling about quantum computing
Forward: features are independent pieces written for Mewburn Ellis discussing and celebrating the best of innovation and exploration from the scientific and entrepreneurial worlds.
Back in November 2020, the UK set off on a voyage of DISCOVERY, with the launch of new industry-led quantum computing project of that name. The project is part-funded by the UK National Quantum Technology Programme, which is investing £1bn in the effort to overcome the technical barriers to commercial quantum computing.
It’s part of a push that aims to ‘place the UK at the forefront of this transformative new technology’, with the ambition of making the UK ‘the world’s first quantum-ready economy’. The projects promise: ‘companies across the country will have access to our first commercial quantum computer’, to be based at the National Quantum Computing Centre in Oxfordshire.
As Andrew Fearnside, a patent attorney at Mewburn Ellis, comments: ‘Prototype quantum computers exist today, but the holy grail for quantum technologies is creating a commercial, scalable quantum computer that can compute indefinitely without errors. It is hoped that the partnerships fostered by the DISCOVERY project will provide the key to overcoming existing technology challenges.’
Why is so hard to achieve quantum computing at scale? Most importantly, because the raw computing materials required for quantum computing are qubits – quantum ‘bits’ that represent data (the ‘zeros’ and ‘ones’ of our current ‘classical’ computers) – and qubits aren’t known for their stability. They act according to the ‘rules’ of quantum physics, which describe how very small objects are built, move around and interact with the outside world. All elementary particles obey these rules, which are difficult to define because they are hidden by the randomness of the uncountable numbers of particles that make up the things around us.
‘If we could focus down on an individual particle, for instance an electron,’ explains Fearnside, ‘its rules would seem very different to the rules governing the object (perhaps an apple) it forms a small part of. In this sense, the particles making up the object are individually “quantum”, but the big object they form a part of is not.’
These quantum rules are regularly described as ‘shocking’, and for good reason. As Niels Bohr, a founding father of quantum theory, once put it: ‘Anyone who is not shocked by quantum theory has not understood it.’
Examples of such shocking behaviour include:
Whereas classical bits can only be a one or zero, thanks to these ‘shocking’ properties a qubit can exist in many states at one time – so zero and one simultaneously. On the plus side, this means a successful quantum computer can give you more bang for your buck – for instance, because each qubit can do many things at once (thanks to superposition) and can coordinate with other qubits instantaneously (thanks to entanglement).
It’s what Fearnside calls (to use a loose analogy) ‘a sort of collaborative processing on an enormous scale’. In fact, British physicist Professor David Deutsch describes quantum computation as ‘a distinctively new way of harnessing nature’ and predicts that it will be ‘the first technology that allows useful tasks to be performed in collaboration between parallel universes’.
Deutsch, as Fearnside explains, believes that when you run a computation on a quantum computer, you run it simultaneously on many instances of the computer that exist in parallel universes. Each instance of the computer does a different part of the computation, and the final collaborative result is shared as the output, measured and defined.
“When you run a computation on a quantum computer, you run it simultaneously on many instances of the computer that exist in parallel universes”
On the downside, qubits have a habit of interacting with their environment, creating what is known as ‘noise’, and this can lead to instability (decoherence) and computation errors. In order for a quantum computer to be commercially viable, it would take qubits made from something that allows them to be grouped closely enough for the useful quantum rules (superposition and entanglement) to work well, but which also allows them to remain stable enough for calculations to be completed without accumulating unmanageable errors.
In the context of a quantum computer, Fearnside explains, keeping qubits on task is ‘like keeping a number of plate-spinning tricks going at once: each qubit is a plate spinning on a stick and you need to keep them spinning all at the same time.’
It’s a tall order, indeed. But like the search for the holy grail, the promise of the prize is enough to keep scientists trying, and four approaches seem to be emerging as leading contenders:
These strategies are yet to prove their worth, but for the organisations involved, it’s all to play for. A successful quantum computer at scale would far outstrip the capabilities of its classical counterparts, which are quickly reaching their limits in terms of the number of component parts they can incorporate and how small those parts can be made.
‘Once reached, commercial quantum computers could speed up scientific discovery in ways that normal computers just can’t manage,’ Fearnside says. ‘From designing new green technology such as batteries and materials, to better medicines or more secure telecommunications, the potential benefits are huge.’
For more news and insights on quantum computing, view our blog. We've also spoken to Mark Saffman of ColdQuanta and Jelmer Renema of QuiX – as part of a series of conversations with leading innovators aiming to make quantum computing a commercial reality.
Written by Caitlin Mackesy Davies
Andrew is a Senior Associate and Patent Attorney at Mewburn Ellis. He works primarily in the fields of telecoms, electronics and engineering, and specialises in quantum technologies, photonics and ion optics. Andrew has extensive experience of drafting and prosecution, global portfolio management and invention capture to secure a commercially valuable IP portfolio. He also conducts freedom to operate analyses and performs due diligence.
Email: andrew.fearnside@mewburn.com
Our IP specialists work at all stage of the IP life cycle and provide strategic advice about patent, trade mark and registered designs, as well as any IP-related disputes and legal and commercial requirements.
Our peopleWe have an easily-accessible office in central London, as well as a number of regional offices throughout the UK and an office in Munich, Germany. We’d love to hear from you, so please get in touch.
Get in touch